The Mary Lyon Centre at MTC Harwell and the MRC National Mouse Genetics Network are delighted to host this event which aims to provide insights into resources available in the UK and funding opportunities for research into rare diseases in the UK and Europe. Academia and industry will come together to discuss how public-private collaborations can be established in the field and how to overcome present and future challenges.
The event is free but places are limited so do register here
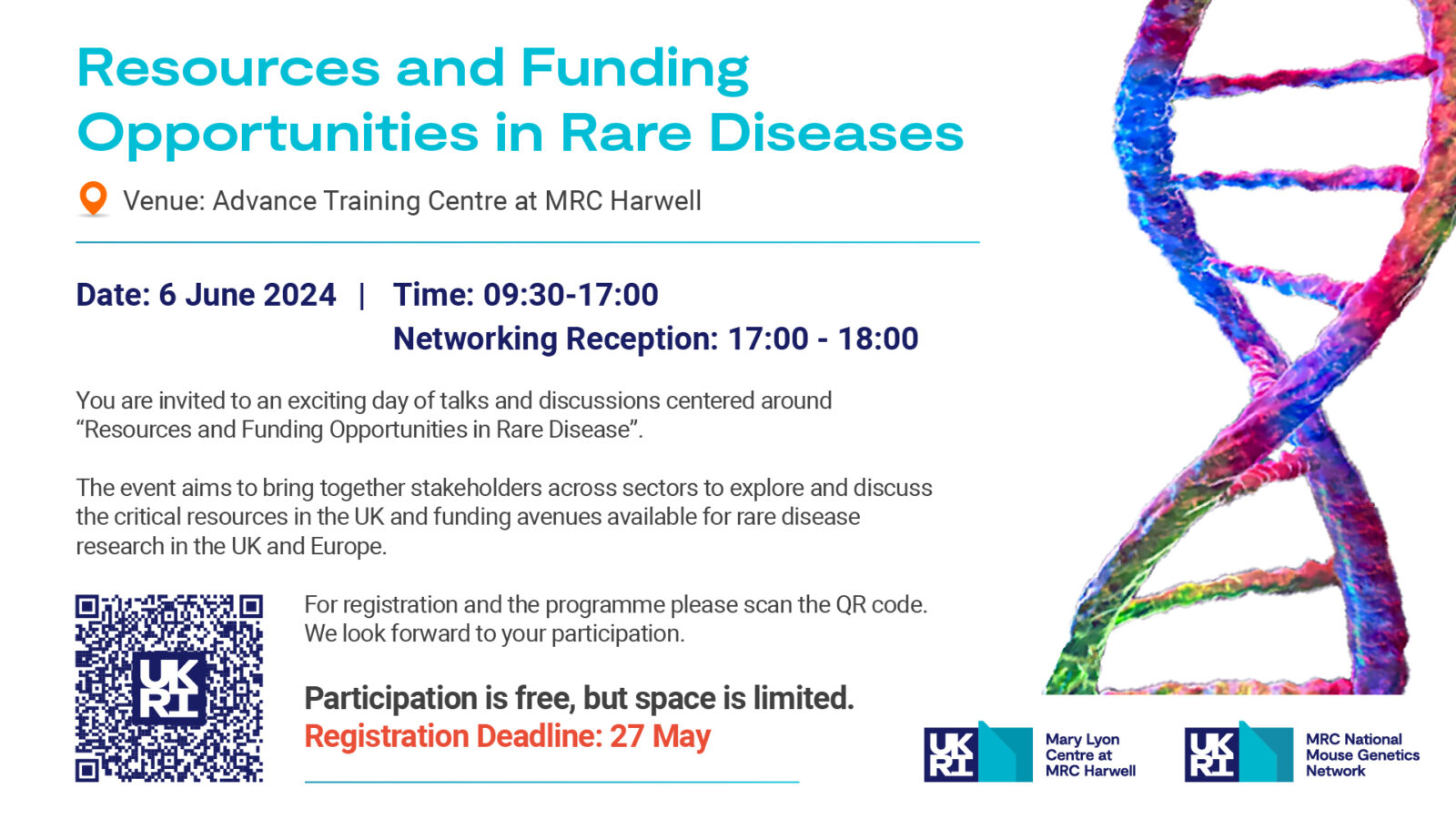