Open and transparent communication about animal research is important for maintaining trust in science and challenging the misconceptions that surround it. Over the last few months, we have been involved in three outreach activities aiming at very different audiences, from primarily younger children making plasticine mice, through talking with teenagers online, to sharing our science in the pub. Through this we can engage with the public in a way that allows us not only to demystify science, but also to listen, learn, and connect with communities across the UK, and hopefully to inspire future generations of scientists.
Pint of Science: From AI to the Microbiome
In May, the MRC National Mouse Genetics Network sponsored a Pint of Science event in Oxford, part of an international festival that brings scientists into pubs to share their research with the public in an informal setting. The event featured talks from Sonia Bains (Head of Phenotyping Technical Development at the Mary Lyon Centre) and Avery Robinson and India Brough (post-docs with Jethro Johnson and Fiona Powrie, respectively, at the Kennedy Institute of Rheumatology, and who are also part of the Network’s Microbiome Cluster).
Sonia spoke about how artificial intelligence and microelectronics are transforming the way we monitor health and understand behaviour, while India and Avery explored the fascinating world of the gut microbiome and how it interacts with the immune system.
“We had an amazing time taking part in Pint of Science and had some great questions! We loved the informal aspect of the event, which promoted a very relaxed atmosphere and more active interactions during question time.” – India
India and Avery are also part of The Gut Florists, an outreach initiative established in 2022 by India and another colleague, Rebecca Jeffery, that aims to reach non-traditional audiences—including festival-goers at Glastonbury.
“Pint of Science was such a rewarding experience. There’s a real hunger out there to understand what scientists do… Being open and accessible not only strengthens public trust but also renewed my own sense of purpose and responsibility as a researcher.” – Sonia
The night was a great success, with tickets selling out weeks in advance and attendees, including the bar staff, complimenting our speakers on their informative and entertaining talks afterwards.
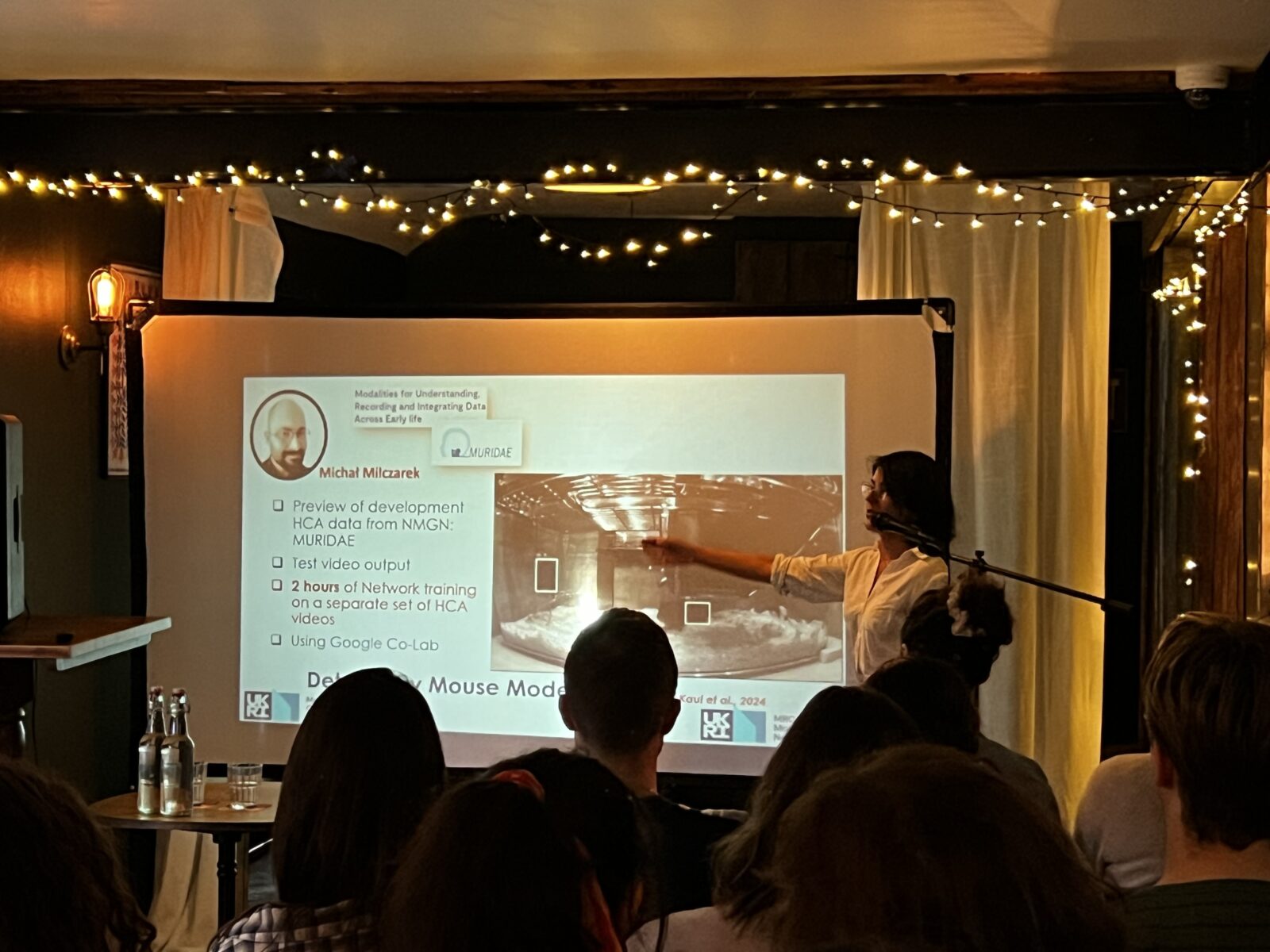
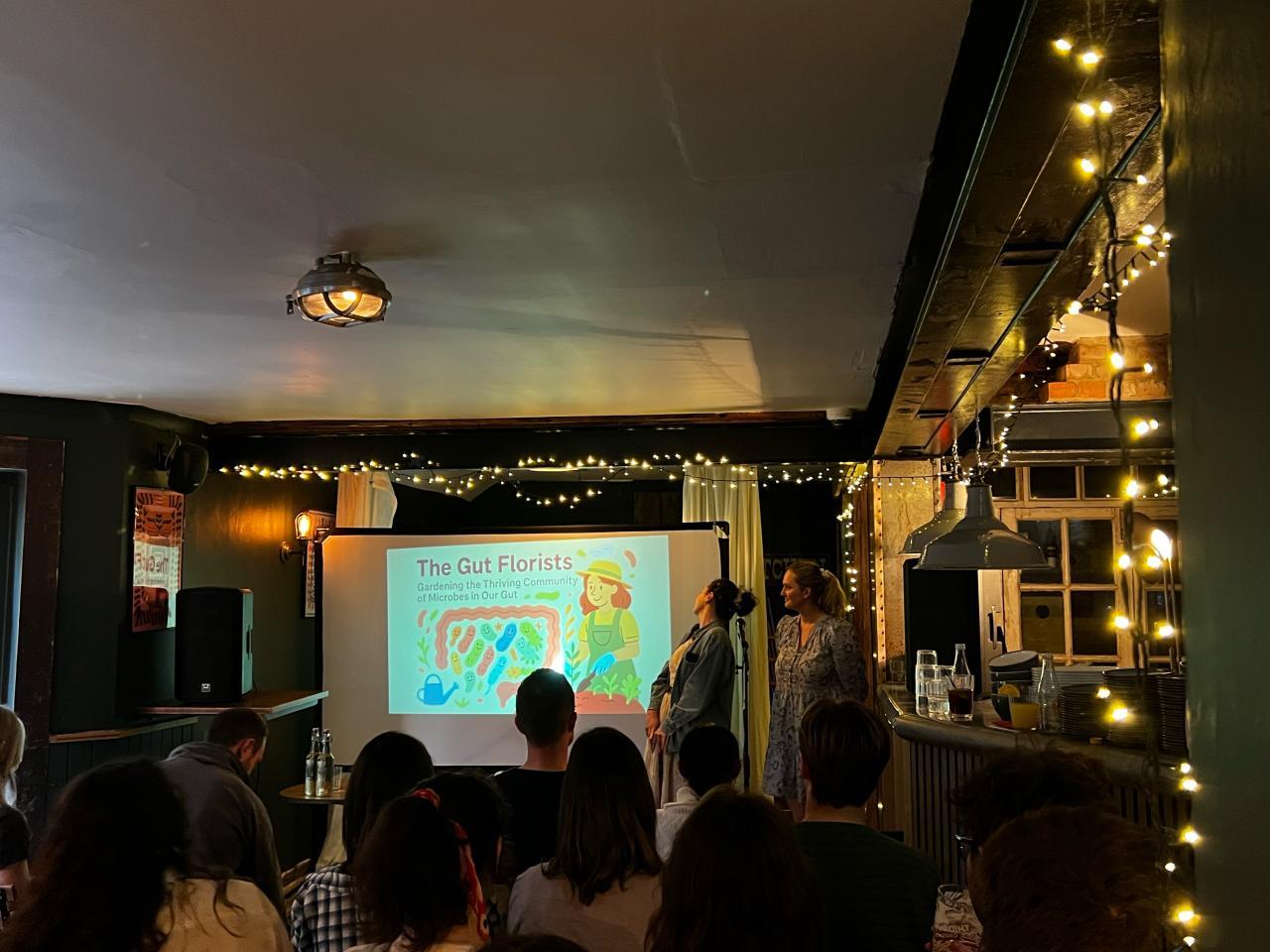
I’m a Scientist: Engaging with Students from Harpenden to the Highlands
Over the last year, ten researchers associated with the Network, including some based at the MLC, have participated in I’m a Scientist, an online STEM enrichment programme that connects school students with scientists through live, text-based chats. The volunteers fielded questions from students across the UK, ranging from Torbay to Tyneside, and from Harpenden to the Highlands. Importantly, most of the schools involved had been identified as priorities based on their distance from their nearest major research higher education institution or on the proportion of their students receiving Free School Meals or Pupil Premium.
Chats were themed around topics selected by the school and covered everything from more general topics like the day-to-day life of a scientist and how to work scientifically to more specific topics like artificial intelligence, genetics, and antimicrobial resistance, all alongside a number of chats specifically about the use of animals in research, a topic that we had encouraged I’m a Scientist to establish.
“I enjoyed answering questions around a scientific career, knowing that I can share perspective and insights into jobs that I would have had no idea existed while I was at school.” – Dale Watt, a Senior Scientific Officer at the CRUK Scotland Centre and member of the Network’s Cancer Cluster.
“It’s a great opportunity to show the kids about real-life work experience, and the realistic side of having a STEM job. They need to learn these from real scientists, not from movies or books.” – Tugba Bulbul Atac, a Data Wrangler at the MLC.
“It was a rewarding experience, and I truly appreciated the opportunity to engage with schools and share insights into science and scientific careers.” – Lisa Leinhos, a post-doc at the University of Oxford and member of the Network’s Congenital Anomalies Cluster.
“I have thoroughly enjoyed taking part in ‘I’m A Scientist’. I wish I had this opportunity when I was at school! It is inspiring to get a glimpse into the way they see and approach science, and their ambitious thinking about potential research studies – our future scientific stars are out there!” – Cathy Fernandes, Professor at the Institute of Psychiatry, Psychology & Neuroscience, King’s College London and member of the Network’s MURIDAE Cluster.
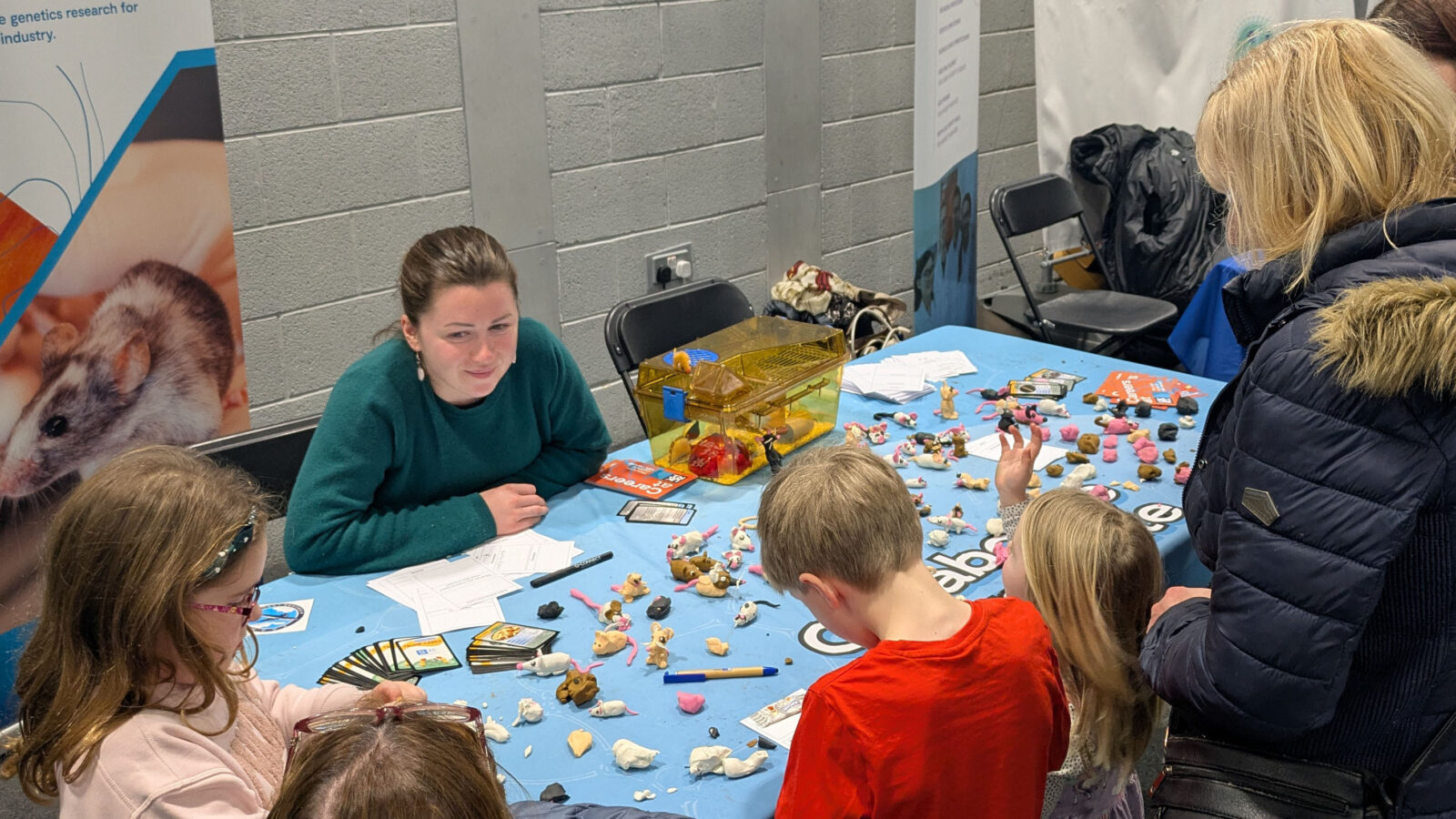
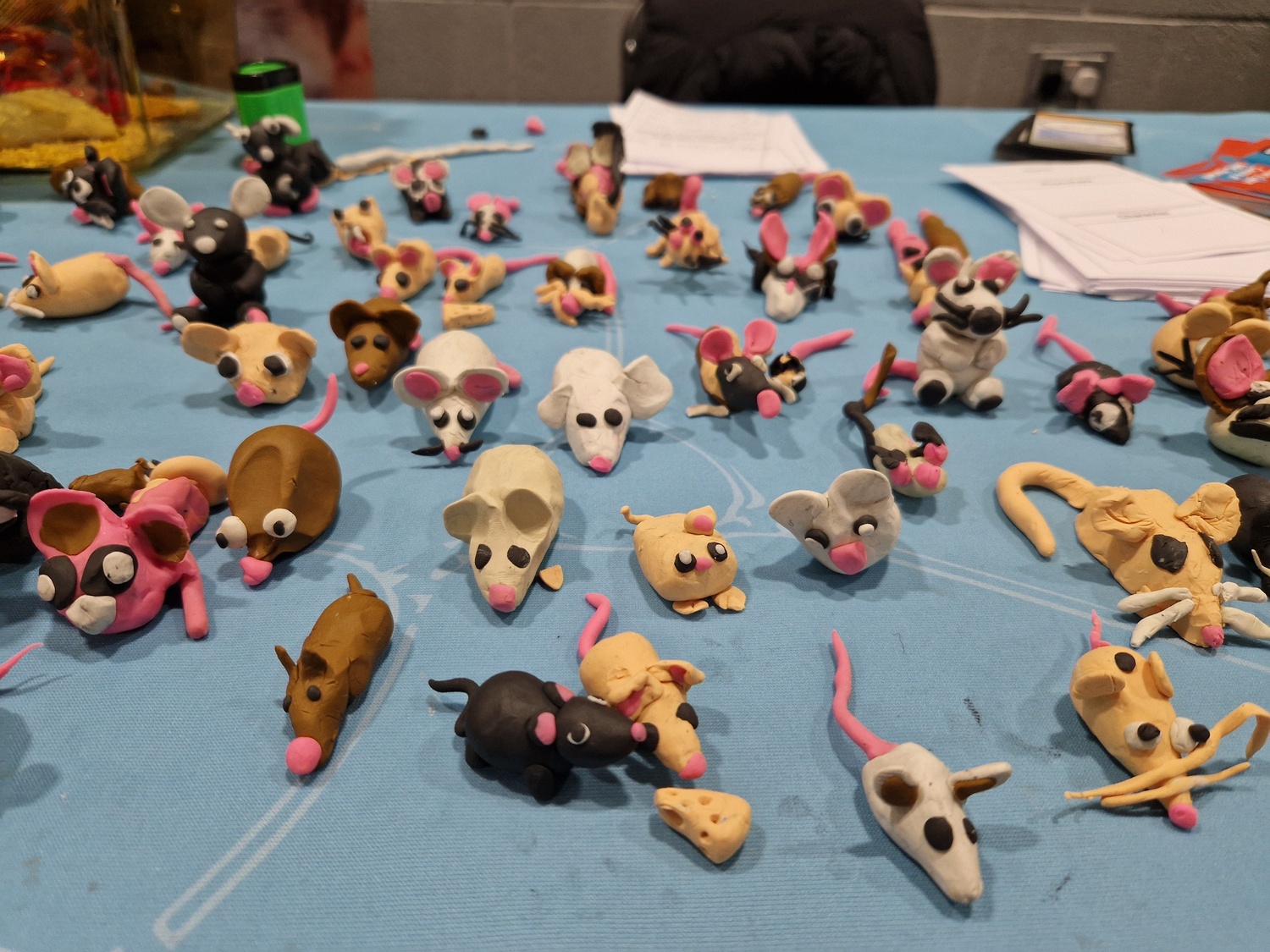
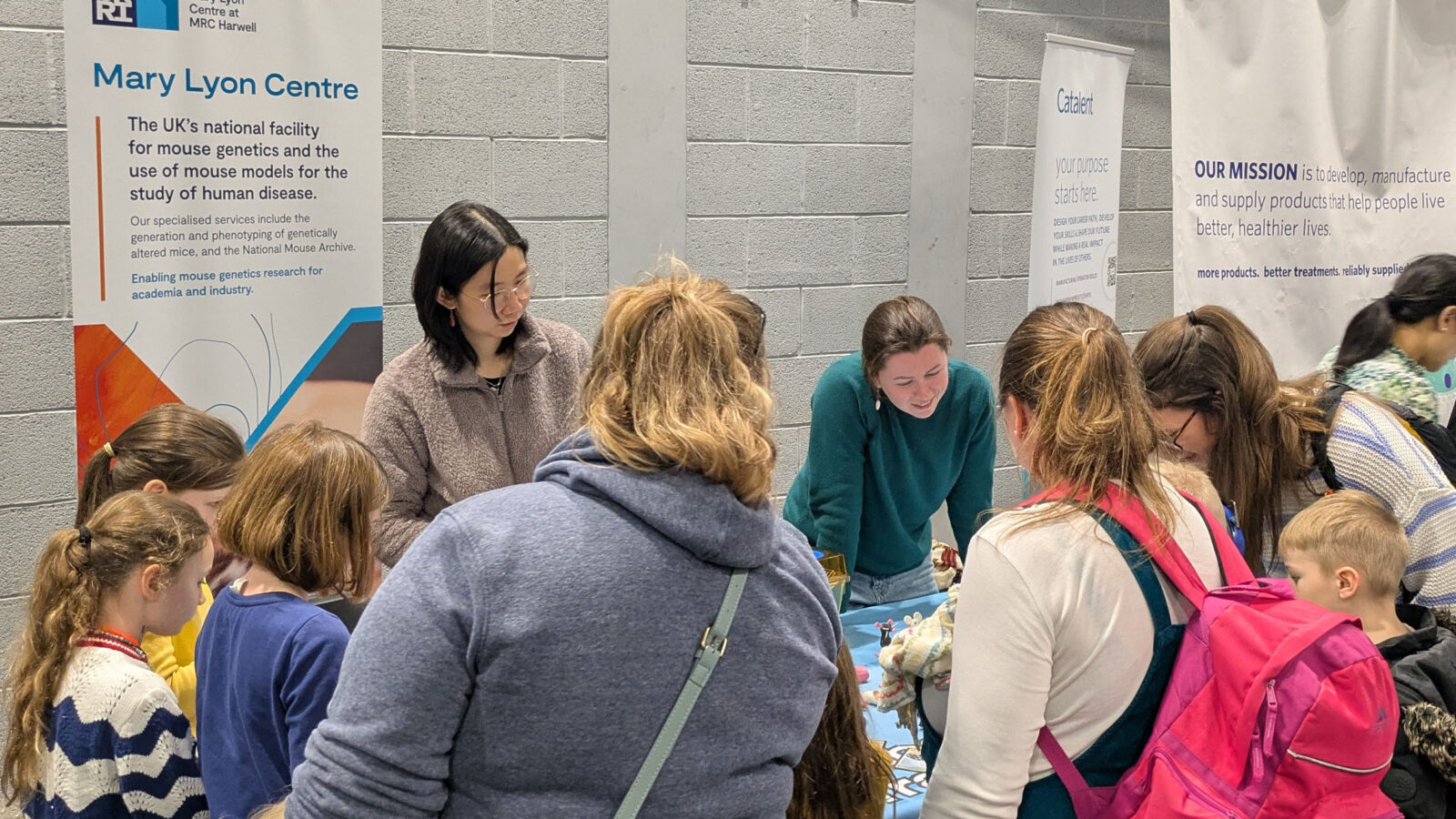
Festival of Tomorrow: Making Model Mice with Children
In February, we took part in the Festival of Tomorrow—a week-long celebration of science, innovation, and the arts, taking place across Swindon, with an estimated 35,000 people taking part in some way. The festival culminated in a vibrant finale event that brought together thousands of attendees, including families, students, and curious minds of all ages.
Our stand at the Festival Finale Discovery Zone featured a hands-on activity where visitors were invited to create their own model mice out of plasticine. This simple yet engaging task opened the door to conversations at different levels depending on the festival attendee, from talking about how big mice are and what they look like, through to where scientists obtain mice, how we care for them, how we make new disease models, and how their use contributes to biomedical science.
Over the course of two days, hundreds of plasticine mice were made and 99 information sheets filled out—each one a small but meaningful connection between science and the public. While talking about how we use mice, the children were encouraged to complete a small card describing their mouse, getting them to think about what disease they might want to model or what they might hope to learn from their mouse.
These public engagement activities reflect our ongoing commitment to making science more inclusive, transparent, and inspiring. Whether through digital platforms, creative workshops, or casual conversations over a pint, our researchers are helping to build bridges between the lab and the wider world. We’re proud of the enthusiasm, creativity, and dedication they have shown.